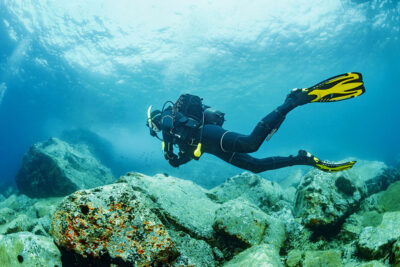
Taking a Deep Dive into Diving
Around the world, millions of individuals over the last century have taken advantage of impressive improvements in diving technology to allow them to explore the various coral reefs, kelp forests, shipwrecks, and other fascinating underwater sites. There is always some associated risk, whether a dive is done recreationally, as an instructor, as a semi-professional, or as a professional technical diver. Any time someone goes below the surface, they are subjected to the same laws of physics, which, if understood improperly, can result in significant injury or death. Additionally, divers are at risk for various forms of underwater trauma from boats, other divers, and aquatic life. According to the Sports and Fitness Industry Association, there are approximately 2.5 million active scuba divers in the United States alone.1 Despite well-known dangers, thousands become scuba certified each year and then go on to perform only very occasional dives, with little interval supervision and a poorly practiced skill set. A suboptimal understanding of the risks associated with diving may subject individuals to the injuries they can experience from improper diving techniques. This article describes the various gas laws that govern underwater physiology and common underwater trauma and mishaps.
History of Diving and Compression Illness
Since the invention of scuba in the 1950s, millions of people have been exposed to greater and greater depths, and with that, the dangers of breathing compressed gas. The physiology of increased pressure on the human body was not widely studied until the construction of two bridges in the United States: the Brooklyn Bridge in New York and the Eads Bridge in St. Louis. Assembling these bridges required the use of pressurized structures known as caissons. These caissons allowed workers to excavate mud down to the bedrock level, where steel pilings could be driven into the rock, allowing for a safe and robust bridge construction above. They were pressurized using a surface-supplied air supply to prevent water from seeping into the caisson as it was excavated. This resulted in the workers, who were exposed to this pressurized air for 12-hour shifts, developing symptomatic decompression illness when they emerged after their grueling shift. A popular trend in fashion at that time was a corset-laced dress that would cause a functional lordosis that became known as the “Grecian bend.” Since low back pain was a common symptom of the decompression illness encountered by these workers, causing them to bend over in pain, these workers resembled the lordotic women, and now the disease is known colloquially as the “bends.”2 Although decompression illness was first described by Robert Hooke when he rapidly depressurized a snake in a rudimentary vacuum chamber, leading to the development of an air bubble behind the eye, medical interest in pressure physiology remained at the hobbyist level of inquiry until the pathophysiology of these caisson workers became more widely known.3
Gas Laws
Henry’s Law
C = kP
C is the concentration of dissolved gas, k is Henry’s law constant, and P is the partial pressure of the gas.
To fully understand the injuries associated with diving, a review of the physics of pressurized and atmospheric gas and the physiology of the diver must be explored. The first one of these is Henry’s law, which states that as the pressure above a gas in solution increases, more of the atmospheric gas will dissolve into the solution. This phenomenon is what is primarily responsible for the development of decompression illness. Nitrogen is an inert gas at one atmosphere, or 760 mmHg , and has little effect on the daily operations of the human body when operating at sea level. However, at depth, the body is subjected to an additional pressure of one atmosphere for every 33 feet (10 meters) in diving depth. This increased pressure on the body encourages the nitrogen being inhaled at pressure to become solvated into the tissues at twice the normal concentration one would expect. If the diver then ascends too quickly, this excess nitrogen moves back into gas form as ambient pressure decreases. The nitrogen gas then bubbles out of the tissues, resulting in microbubble formation, which showers the joints and small blood vessels, leading to the symptoms of decompression illness. This phenomenon is easily visualized when a can of soda is opened. The foaming, erupting beverage provides a visualization of the carbon dioxide coming out of solution now that it is subjected to a decreased pressure.4
Dalton’s Law
Ptotal = P1 + P2 + P3 + P4 + P5….+ Pn
Another concept important to diving physiology is Dalton’s law, which states that the partial pressure of each component of a gas mixture is additive to the total pressure of the mixture. In a gas mixture at 100 mmHg , a 10% oxygen concentration equals a partial pressure of 10 mmHg. In hypobaric and hyperbaric physiology, this concept is of extreme importance, as the human body is not responsive to a concentration of oxygen but rather the partial pressure of the oxygen being inhaled into the lungs and then subsequently exposed to the hemoglobin of red blood cells moving through the pulmonary vasculature. For example, regular atmospheric oxygen concentration on Earth is 21% oxygen or 160 mmHg. If a person were to ascend to an altitude of 5,500 meters or 18,000 feet, atmospheric pressure would be roughly half of what is found at sea level. Therefore, the person then would experience a partial oxygen pressure of 80 mmHg. If brought to that altitude quickly without any acclimatization, the patient soon would become hypoxic. Conversely, if a patient dives to a depth of 10 meters either in a hyperbaric chamber or a body of water and then is given a gas mixture of 10% oxygen, they would be breathing oxygen at 160 mmHg. They would have no symptoms of hypoxia despite breathing a gas mixture of half of the amount of concentration of oxygen that usually is found at sea level.5
Boyle’s Law
P1V1 = P2V2
P1 = first pressure, P2 = second pressure, V1 = first volume, V2 = second volume
Boyle’s law demonstrates the inverse relationship between pressure and volume. As the volume of a gas decreases, pressure increases, and vice versa. A gas that is subjected to a pressure of two atmospheres would occupy 50% less volume than the same amount of gas at sea level. A bubble of gas at three atmospheres would occupy only 33% of the volume at sea level, and at four atmospheres, it would occupy only 17% of the total volume at sea level. We see Boyle’s law in effect when a diver is going down to depth. The gas trapped in physiological spaces of the body, including the sinuses, the inner ears, and the mask, can result in an injury called barotrauma.5
Pascal’s Law
Lastly, Pascal’s law states that the pressure on a fluid body is distributed equally. This is why one atmosphere, which is equivalent to 14.7 pounds per square inch, does not crush the human body even at pressures of four to five atmospheres or more, which are encountered when diving beyond 30 meters.6
Diving injuries due to the effects of the gas laws are categorized primarily under Henry’s law, Dalton’s law, or Boyle’s law. These can be categorized further by separating the laws implicated during descent, depth, and ascent. Injuries of descent are due to Boyle’s law. Injuries at depth are due to Dalton’s law. Those of ascent are dictated by both Henry’s law and Boyle’s law. The various injuries encountered during these different phases of diving are described in the following sections.
Diving-Related Injuries and Illnesses
Non-Pulmonary Barotrauma
During descent, trapped gas inside of a diver’s ears, sinuses, gastrointestinal tract, and lungs occupies a smaller volume as pressure increases due to Boyle’s law, as described earlier. Divers first notice this with a mildly annoying sensation inside their ears or sinuses. This progresses to outright pain as the trapped air in these spaces contracts, subjecting the surrounding tissues and bone to a vacuum-like force. Divers are trained to relieve this pressure by equalizing the spaces through a Valsalva maneuver. The most generally taught Valsalva maneuver is to pinch one’s nose and then blow into it, which allows for equalization of air from the nasal passages into the sinuses as well as air in the oropharynx to enter the eustachian tubes and the middle ear.7 If a diver continues to descend without performing an equalization maneuver, the reduction in volume will continue to cause increased pain and eventually damage to surrounding structures. Blood vessels in these spaces eventually will be disrupted, filling them with fluid and relieving pain as the pressure equalizes.
Other areas of the body that commonly fill with air are subject to this effect. For example, air trapped inside crowns, fillings, or other dental work can cause tooth pain as this air pocket is reduced. However, gastrointestinal gas shrinking in size typically has little consequence for most divers because of the ability of the gastrointestinal tract to accommodate volume changes and decompress excess air relatively quickly.
A special kind of barotrauma is encountered if a diver straps on a mask at sea level and then rapidly descends to depth without equalizing the pressure behind the mask. As the diver descends, the volume of air inside the mask decreases. This creates a negative pressure sufficient to burst capillaries of the conjunctiva, skin, and periorbital area, resulting in significant subconjunctival hemorrhage and periorbital ecchymosis. Although sometimes impressive in its presentation, a mask squeeze usually is of little medical consequence because most patients go on to fully recover. Should a diver become a victim of a sinus or middle ear squeeze that is of a sufficient magnitude to cause damage, general recommendations include cessation of diving operations for the next 72 hours, supportive care for pain control, nasal packing as needed for epistaxis, and otolaryngologist (ENT) specialist referral if a tympanic membrane rupture is noted.8
Pulmonary Barotrauma and Air Emboli
One of the most severe diving injuries is caused by divers ascending quickly without exhaling, causing pulmonary barotrauma. Recalling Boyle’s law, gas breathed in two atmospheres occupies 50% less volume than at one atmosphere. This rapid expansion of volume can burst alveoli and allow the introduction of air into various spaces. These include the potential space surrounding the lung parenchyma (a pneumothorax), the mediastinal and subcutaneous space (pneumomediastinum and subcutaneous emphysema), and the pulmonary venous system (air emboli).
Air emboli transit the vasculature in the same distribution as normal blood flow. Once this air reaches the left side of the heart, it is mixed in the left ventricle and proceeds to shower the tissues with fine air bubbles via the arterial system. These gas bubbles, or air emboli, can produce impressive symptomatology resembling stroke, myocardial infarction, and other manifestations of ischemia. By far, stroke is the most likely pathophysiology to be seen due to the large amount of cardiac output sent to the brain, the brain’s small arterial beds, and its low metabolic reserve. Symptoms of air emboli occur very quickly after the diver has surfaced, differing from decompression illness, which has a more delayed presentation. The prevention of air emboli is an essential aspect of diver training. To avoid pulmonary barotrauma, which causes the emboli, divers are instructed to never hold their breath during ascent. If an unconscious diver has an open glottis, they also will passively exhale the air when brought up from depth, hopefully negating the effects of Boyle’s law on the air inside their lungs. That said, developing an air embolus is always a consideration during any diver rescue from depth. The change in pressure required to rupture the alveoli is relatively small, and air emboli have been documented in divers who have held their breath and ascended as little as three feet.9
The treatment for an air embolus includes 100% oxygen, if available, and prompt treatment in a hyperbaric chamber to diminish the size of the offending air embolus. Transportation to the hyperbaric chamber should be done to minimize the patient’s exposure to altitude, but the cruising altitude of most helicopter operations generally is safe. Prompt evaluation by a hyperbaric specialist is necessary for these patients since their stroke symptoms will persist until a hyperbaric chamber dive can be performed. Thrombolytics typically given in ischemic strokes will not have any effect on the offending air embolism and carry a significant unnecessary risk of hemorrhage.10
Reverse Squeeze
Boyle’s law phenomenon is the reverse squeeze, which can occur in the same non-pulmonary air-filled body areas affected by a squeeze. This is related to over-distention of the air-filled space as the volume of gas expands during ascent. The middle ear and sinuses are especially susceptible if the diver ascends too rapidly without allowing pressure equalization in those spaces. During the ascent phase of the dive, the diver may have epistaxis, bleeding from the ears, or hearing impairment due to blood trapped behind the tympanic membrane. The pressure differential in the middle ear can build to a point where the tympanic membranes rupture, ultimately equalizing pressures. It is imperative to screen divers who have experienced signs and symptoms of reverse squeeze for any signs and symptoms of air embolism. It also is essential to monitor these patients for signs of decompression illness, since reverse squeeze may be indicative of a rapid ascent. Treatment for reverse squeeze generally is supportive, such as nasal packing for epistaxis and pain management. If tympanic membrane rupture occurs in dirty water, antibiotic prophylaxis also should be considered.11
Oxygen Toxicity
Most recreational divers breathe a mixture of either compressed atmospheric air or an enriched oxygen and nitrogen mixture known as nitrox. Because of Dalton’s law of partial pressure, there are two conditions affecting divers at a depth with which divers and providers should be familiar. The first is oxygen toxicity, which develops in most people when oxygen reaches a partial pressure of 1.4 atmospheres. This can occur at a depth of 187 feet at 21% oxygen, the same concentration of oxygen in the earth’s atmosphere.12 Oxygen toxicity can present with a spectrum of features, including paresthesias, vision changes, tinnitus, nausea, tremors, cough, chest discomfort, and eventually will trigger seizures when a sufficiently toxic dose is accumulated.13 Because of the dangers of suffocation and the lack of support found in deep water, a seizure underwater can be life-threatening not only to the diver affected but also to dive buddies and potential rescue personnel. Fortunately, the seizure activity generated by oxygen toxicity at depth resolves quickly after the patient has ascended to more shallow depths. Should an oxygen toxicity event occur at depth, the diver should ascend (or be helped to ascend) to a safe depth slowly to avoid decompression illness or air embolism. Ideally, the rescuer should ensure the diver’s regulator remains in their mouth. A more rapid ascent may be required if the diver is not breathing. However, with all rescue missions, the safety of the rescue crew must be considered.
Divers who plan to dive to a moderate depth of 30 meters or less but plan to dive for a prolonged period often will use an enriched oxygen gas mixture known as nitrox. Nitrox, with its lower nitrogen concentration, results in a lower partial pressure of nitrogen when compared with atmospheric air when breathed at depth. Because of the increased oxygen concentration in this gas mixture, breathing nitrox at depth means that the body is exposed to a greater partial pressure of oxygen for a given depth when compared to atmospheric air and can result in oxygen toxicity at a shallower depth of onset. Thus, nitrox can be dangerous for deep dives, and, as previously mentioned, an underwater seizure caused by oxygen toxicity is a critical event with significant morbidity and mortality.14
Nitrogen Narcosis
The second gas-related pathology that occurs at depth is nitrogen narcosis. Colloquially known by divers as “martini’s law,” the effect of nitrogen narcosis is roughly equivalent to one alcoholic beverage for every 10 meters the diver has descended while breathing air. Therefore, at 200 feet (about 61 meters), nitrogen narcosis provides the equivalent sensorium of six alcoholic beverages. This can be debilitating and disorienting, especially in the cold, dark water often encountered at these depths. Although most recreational divers do not descend to these depths, nitrogen narcosis is of considerable concern for professional divers attempting to work with power tools in dangerous environments with numerous industrial hazards, such as oil rigs. Unless the diver is equipped with a special radio, it can be challenging to recognize when a person is experiencing this condition. A solo diver can become blissfully unaware of the danger they are in and can continue to descend to greater depth, which only exacerbates the problem they are experiencing and ultimately can result in fatality. Hence, the colloquial name for this illness in the diving community is “rapture of the deep.”15 It is crucial to start a slow and deliberate ascent at the first sign of even mildly incapacitating nitrogen narcosis.
All beginner and most recreational divers breathe compressed atmospheric air, composed of 21% oxygen, 78% nitrogen, 1% argon, and a few other trace gases in various quantities. Additional gas mixtures in use primarily in industrial diving are trimix and heliox. Trimix attempts to solve the issue of the oxygen toxicity of nitrox by adding helium to the mix. This is an inert gas with no metabolism in the body and significantly less neurocognitive impact. Trimix also has a lower amount of nitrogen and can prevent nitrogen narcosis. While breathing trimix, divers can dive deeper with a lower risk of oxygen toxicity and nitrogen narcosis. Since helium remains extremely inert even at tremendous pressure, divers can explore depths of up to 200 meters or more without the effects of nitrogen narcosis or oxygen toxicity. Heliox minimizes the risks of nitrogen narcosis and decompression sickness by eliminating nitrogen and replacing it with more helium. Both trimix and heliox are expensive when compared to nitrox or atmospheric air and, therefore, typically limited to professional-level divers only.16,17
Decompression Sickness
Outside of middle ear barotrauma, the vast majority of diving-related severe injuries occur during ascent. While divers are at depth, they are increasing the total body concentration of various gases because of the increased pressure dissolving gas into their tissues in a solute form. This includes all gases, especially the predominant gas in the air: nitrogen. If the diver ascends slowly, this nitrogen has a chance to be released gradually from the body through respiration. If the diver ascends quickly, nitrogen can leave the tissue abruptly, resulting in bubble formation, which finds its way into joints, subcutaneous tissue, the neurological system, and the cardiopulmonary system. This sudden off-gassing of nitrogen is similar to the release of carbon dioxide from a warm bottle of soda that is opened and allowed to rapidly equilibrate to ambient pressure. The same mechanism occurs with nitrogen inside the tissues of the body during ascent.
Depending on the magnitude of the symptoms, decompression sickness (DCS) can be classified as type 1 or type 2 DCS. Type 1 DCS involves only the joints and/or skin. Common joints involved are the knees, shoulders, and hips. Patients commonly develop symptoms within two to three hours after the dive and experience a dull soreness. (See Table 1.) This begins to worsen over several hours in the aforementioned joints. Gas bubbles in the skin can result in a lace-like rash known as cutaneous marmorata. (See Figure 1.)
Table 1. Types of Decompression Sickness and Their Clinical Features |
||
Classification |
Clinical Features |
Comments |
Type 1 |
|
|
Type 2 |
|
|
Figure 1. Cutis Marmorata in a Patient with Decompression Sickness |
![]() |
Source: Huckfinne, CC0, via Wikimedia Commons. https://creativecommons.org/pu... |
Type 2 DCS is associated with decreased sensation, weakness, or altered mental status. This is due to the formation of gas bubbles proximate to neurovascular structures and the subsequent inflammatory cascade that impairs the function of those structures. When the burden of gas formation inside the body is so high that it begins to overload the pulmonary arterial system, bubbles can transit to the pulmonary venous side and shower the cardiopulmonary system and brain. This can effectively cause a pulmonary air embolism, cardiac ischemia, or stroke.18,19
Because of the delay in the onset of symptoms, which typically is seen, divers may confuse the initial symptoms for an overuse injury during the dive or during activities preparing for the dive that day. Taking a good history provides vital information to help differentiate decompression sickness from other musculoskeletal injuries. This complete dive history includes: how many dives the diver had performed that day, their total bottom time, the maximum depth they descended to, their rate of ascent and any decompression measures, the type of work or recreation being done during the dive, any symptoms of other divers in the group, and any other abnormalities related to the diving activity. Many modern divers dive with special computers equipped with a barometer informing them if they are outside their safety zones. However, the average recreational diver may need access to this equipment and may rely on a hired dive guide to provide them with a safe diving experience.
The definitive treatment for decompression sickness is a hyperbaric chamber. Ideally, evacuation can occur via ground transportation to avoid unnecessary additional changes in altitude; however, evacuation via air transport in the cases of severe decompression illness is warranted if it will expedite access to treatment. In air transport cases, the aircraft should fly at a low altitude or below cabin altitude to prevent worsening decompression illness. Ascent reduces the pressure on the body and could encourage more of the solvated nitrogen to come out of the tissues. If available, supplemental oxygen should be administered since 100% oxygen creates a diffusion gradient for the nitrogen in the body and encourages the off-gassing of the free nitrogen circulating in the bloodstream.20,21
Once at the hyperbaric facility, the patient is placed into a hardened chamber and pressurized to depth. This forces the bubbles inside the diver to dissolve back into the tissue and allows for a slower, safer off-gassing of the bubbles. The hyperbaric chamber also has a secondary protective effect against the inflammatory cascade that the tiny bubbles cause within the diver’s tissues. Decompression illness is ideally treated within 24 hours. Hyperbaric treatment of divers often demonstrates rapid symptom improvement as the patient reaches treatment depth.22 The duration and depth of the hyperbaric chamber treatments are dictated by a series of dive tables published by the U.S. Navy. Depending on the severity of the decompression illness, one dive or multiple dives may be required for symptom resolution. Consultation with a hyperbaric center or the Divers Alert Network (DAN) is highly beneficial for the treatment of all known or suspected decompression illness casualties.23,24
Drowning
Drowning is one injury that should be considered at all dive phases. Drowning occurs when the diver asphyxiates due to the inability to ventilate because they are underwater without an adequate air source. In diving, drowning can occur at all phases of the dive. For all drowning victims, if the victim is pulseless, rapid recovery followed by immediate cardiopulmonary resuscitation (CPR) with rescue breaths is the best modality of treatment. While stabilizing the patient, it is important to evaluate for signs as to why they may have drowned, including trauma, faulty or improper equipment, attack or poisoning by aquatic life, an underwater seizure, intoxication, hypoglycemia, and other causes. All dive team members, including physicians, should be familiar with the hand motions described by dive instructors. If a diver is found in distress, the diving party can rescue them quickly.
Of special consideration among free divers, swimmers, and snorkelers are shallow water blackouts. This occurs when a person hyperventilates before diving underwater, which is related to two potential mechanisms: reflexively hyperventilating while submerged in cold water or deliberate hyperventilation to build up a large reserve of air before going underwater. Hyperventilation removes a large quantity of circulating carbon dioxide, reducing the body’s trigger to breathe. The induced hypocapnia from hyperventilation reduces respiratory drive and delays the time to trigger a breath, allowing a diver to become hypoxic without an urge to breathe. This phenomenon can be expedited with strenuous activity or diving in cold water. As the name suggests, it is important to note that shallow water blackouts can occur in very shallow water, including swimming pools, beaches, and other areas where people may be free diving in relatively low depths. Treatment is rapid removal of the diver from the water, initiation of rescue breaths, and CPR if the victim is pulseless.25
Traumatic Injuries
The realm beneath the waves is an area rich with mechanisms that can cause trauma, most of which are human-made, but some are natural. Divers trying to dive in rough but shallow water may be thrown against rocks, resulting in blunt injuries. A popular recreational activity, especially in warmer areas, uses a tension-bound projectile weapon known as a spear gun to fish for aquatic life for individual consumption. There are reports of humans being impaled by these spears, causing significant life-threatening injuries.26 Boat propellers are another common cause of trauma in divers, which is why it is recommended that a dive flag be placed anytime divers are present to alert boat operators of the hazards of divers in the area. Boat propeller traumas can be grievous and result in rapid hemorrhage and death prior to the diver even being able to be placed into the boat to seek definitive medical care.27,28 Divers also can become entangled in lines from anchors, fishing nets, or any other sort of device that is anchoring a surface structure to the water, such as a buoy. These hazards are especially pertinent in murky water because of poor visibility. Large aquatic animals, such as giant grouper, sharks, dolphins, manta rays, and whale sharks, can cause sufficient blunt trauma to incapacitate a diver. Lastly, various aquatic life can cause life-threatening bites or other injuries through various mechanisms. Although sharks come to mind, toothed fish, such as barracuda, moray eels, and other aquatic predators, also can cause painful, potentially incapacitating bites if provoked. Famously, large stingrays can deploy a barb sizable enough to result in pericardial tamponade or other organ injury, as was seen in the death of Steve Irwin in 2006.29
Treatment of diving trauma should be approached in the same manner as any other trauma, first ensuring a safe scene for any rescuers involved. Rescuing a diver who has experienced trauma can be made more complicated if they need to be extricated from the water first. This is especially difficult in water with poor visibility, cold water, or swift currents. As previously mentioned, there is always the possibility of either the victim or the rescuers developing decompression illness or air emboli if the ascent is rapid. A stepwise approach to evaluation and resuscitation should occur once the diver has been brought to the surface. One approach is the MARCH mnemonic — first addressing any areas of massive hemorrhage, then moving on to evaluate the airway, respirations, circulation, and then assessing for hypothermia or hyperthermia.30 If the casualty is unconscious but still breathing, stabilizing their cervical spine while removing their diving equipment, administering supplemental oxygen if available, and tending to their wounds while waiting for transport to arrive is an excellent place to start. The diver also should be dried off and kept warm to prevent hypothermia. Alternatively, if the event occurs in hot weather, excess gear should be removed, and the diver should be kept out of direct sunlight, if possible, to prevent hyperthermia. Most recreational dive boats do not have a sufficient medical kit to treat major diving injuries, so contacting appropriate sea rescue authorities should be performed as soon as possible. Severe dive injuries should be transported to a trauma center, preferably one with hyperbaric capabilities. Minor traumatic injuries have the potential to become infected, and antibiotic coverage, particularly for penetrating wounds or animal bites, is prudent.
Hypothermia
Hypothermia is of significant concern for any water activity because cold water can cause a rapid drop in core body temperature. Humans lose their body heat in four ways: radiation, conduction, convection, and evaporation. Radiation is transferring body heat via infrared radiation into the surrounding environment. Conduction occurs when heat moves down a gradient from an area of high heat to low heat. Convection, the mechanism most relevant to divers, occurs when a cool gas or liquid moves over the body. Lastly, evaporation is the loss of heat that occurs when a person sweats due to heat loss in the vaporizing liquid.31
The physiological response to hypothermia begins around 35°C, where the Swiss Hypothermia Classification System (HT) begins. The HT system provides a scale at which various predictable symptoms begin and end relative to core body temperature. These are found at 35°C to 32°C for mild (HT-1), 32°C to 28°C for moderate (HT-2), 28°C to 24°C for severe (HT-3), and < 24°C represents profound hypothermia (HT-4/5).32 Since individuals can have somewhat varying responses to hypothermia, there are limitations to these classification systems. However, they can serve as a good guide in the field where continuous temperature monitoring is limited, and providers must rely on physical examination findings. Some of the gross physical exam findings of the four stages of hypothermia are described in Table 2.33
Divers try to ward off hypothermia by using either wet or dry suits to provide a barrier to preserve their core body heat. Wet suits trap a thin layer of water between the skin and the neoprene suit. Body heat warms that thin layer of water, creating a layer of insulation against the cooler water around them. Wet suits can be cold initially, but as the diver moves and continues their dive, this layer of water warms up, resulting in a comfortable experience. For frigid water, a dry suit may be more appropriate. Because of the surrounding cold, wearing insulated clothing to take advantage of the body’s heat release, as well as a possible electric heating system inside of the dry suit, may be necessary if the diver is in particularly cold water or dives for a longer duration. One small study found that skin temperature was significantly warmer after cold water dives in dry suit wearers compared to wet suit wearers.34
As noted in Table 2, shivering is one of the first signs of hypothermia. If a diver starts to experience shivering, they should consider aborting their dive to allow appropriate time to resurface safely and minimize further cold exposure. Ascending while shivering also has the advantage that shallower water generally is warmer than the cooler water found at depth. If early hypothermia goes unaddressed, it may progress to confusion, which can be a significant danger underwater.
Table 2. Swiss Hypothermia Staging System |
||||
Stage |
1 |
2 |
3 |
4 |
Symptoms |
Awake and shivering |
Altered and not shivering |
Unconscious and not shivering |
No vital signs found |
Severity |
Mild |
Moderate |
Severe |
Profound |
Core Body Temperature |
32°C to 35°C |
28°C to 32°C |
24°C to 28°C |
< 24°C |
Adapted from: Paal P, Pasquier M, Darocha T, et al. Accidental hypothermia: 2021 update. Int J Eviron Res Public Health 2022;19:501. |
For patients with moderate hypothermia (HT-2/3), prompt external rewarming is necessary but should be performed with some precautions, especially in the field environment where medical resources are limited. Rewarming patients in moderate hypothermia encourages vasodilation in the peripheral vasculature, where the warm blood begins to perfuse the cooler extremities, causing the core body temperature to begin dropping again in a phenomenon known as core temperature after-drop. This is most important when the patients are on the threshold of moderate and severe, where cardiovascular collapse due to contact with the now colder blood may occur. This can be prevented by initially warming the core as opposed to the peripheral tissues in patients with HT-2/3 hypothermia.35
For HT-2/3 patients being removed from watery or submerged environments, another phenomenon known as circumrescue collapse can occur during and just after removal from water. This is caused by the decrease in the hydrostatic pressure from the water previously surrounding the victim’s body, allowing blood to return to the cold-dependent areas and cooling the heart upon its return to the core, not unlike core temperature after-drop. Keeping the patient horizontal and warming the core after rescue can mitigate this phenomenon. Additionally, the patient should avoid significant physical efforts, which can encourage premature peripheral perfusion and precipitate after-drop.36 Ensure that all hypothermia casualties are placed into an environment that prevents them from becoming cold again. External heat sources should be applied to the axilla, chest, and back. If using commercial chemical heat packs, although effective when applied to the axilla and groin, care should be taken to avoid pressure injury or burn, especially in patients with no or a low state of consciousness.37 The ideal rate of active rewarming is 1-2 degrees C/hour.31
In-hospital hypothermia treatment depends on the severity of the patient on arrival, what interventions already have been initiated, and the facility’s capabilities. For HT-1 patients, dry clothes, external rewarming, and physical activity may be all that is necessary. Patients in HT-2 or early HT-3 will benefit from warmed intravenous fluids and heated high-flow nasal cannula in addition to the interventions described earlier. If a patient is hypotensive on arrival, be cautious administering vasoactive medication, as hypothermia causes vasoconstriction. Adding vasopressors may not yield improvement in blood pressure and risks causing arrhythmias. For HT-3 and HT-4 patients, more invasive rewarming methods may be required, such as intrathoracic warm fluid lavage with chest tubes, central venous rewarming catheters, or extracorporeal membrane oxygenation (ECMO) if available.38,39
Envenomations
Beneath the waves, one can find incredibly diverse environments and some of the most spectacular animals on the planet. However, numerous dangerous and venomous creatures can be found in these environments, of which physicians who care for divers should be aware.
Jellyfish
Ubiquitous throughout the world’s oceans, Syphozoans, or jellyfish, are a common sight in many waters. This primitive but efficient organism ensnares prey using long, thin tentacles equipped with tiny nematocysts, which act like a small poison dart, immobilizing prey. Some species have nematocysts large enough to puncture human skin, creating reactions that range from mild dermatitis to severe pain and systemic toxicity, and in the case of the most dangerous examples, cardiac arrest. The class Cubozoa, or box jellyfish, is the group most often responsible for fatal reactions in Australia, Indonesia, New Guinea, and surrounding waters.40 (See Figure 2.) There are documented cases of fatal box jellyfish stings in the Gulf of Mexico from the species Chiropsalmus quadrumanus. Smaller box jellyfish also can produce an unpleasant sympathomimetic toxicity known as Irukandji syndrome, which is characterized by nausea, tremors, tachycardia, hypertension, and even T-wave inversions with elevated troponin levels. Although relatively rare for North American providers, Irukandji syndrome has been documented in the Gulf of Mexico and Florida.41 Although more common in tropical waters, it is possible to encounter a box jellyfish anywhere in warm salt water. Most jellyfish stings are painful but not harmful. Common species, such as genuses Chrysaora and Cassiopea members, can be more painful depending on the species encountered but usually are not life-threatening. As with all stinging organisms, anaphylaxis is of significant concern, especially in individuals with repeat exposures or a history of anaphylactoid reactions to other toxins.40
Figure 2. Box Jellyfish |
![]() |
Source: Guido Gautsch. Wikimedia Commons. https://commons.wikimedia.org/wiki/File:Avispa_marina.jpg. |
Care should be taken to remove these individuals in open water to ensure that the rescuers are not injured when placing them into a boat or another dry craft. It is important to remember that stings can occur both in and outside the water; all that is required is contact with the nematocysts. Professionals who spend significant time in contact with the ocean, such as commercial fishers, lifeguards, dive instructors, and pearl divers/farmers, should know the signs and symptoms of a jellyfish sting and have the recommended first aid to treat stings.
Fortunately, the immediate first aid treatment modalities are the same for all jellyfish. Deactivation of the nematocysts is paramount because they will continue injecting painful and potentially dangerous venom. Three proven treatment modalities exist: immersing the site in hot water, soaking the area in acetic acid, or applying a thick paste of water and baking soda.42 Should these techniques not work, the treatment is largely supportive to include nonsteroidal anti-inflammatory drugs (NSAIDs) and symptomatic management. For severe box jellyfish envenomations, an anti-venom is available in Australia only.43
Commensal aquatic organisms of the order Siphonophorae are another type of cnidarian, which are very similar in appearance to most jellyfish but are a colony of smaller individuals with different specialized morphologies to assist the colony as a whole. Like the Hydrozoans, they have dart-like nematocysts, which can puncture human skin, resulting in severe reactions and occasionally death. The most encountered siphonophore of medical significance is the Portuguese man o’ war, Physalia physalis, a large creature that can grow to impressive sizes. (See Figure 3.) Patients who are stung by these describe a severe burning sensation, and due to the large size of these animals, swimmers who get caught up in their long tentacles are at significant risk for excruciating, debilitating stings, which can result in drowning due to the severity of the pain or systemic inflammatory responses. These organisms tend to “bloom,” with thousands of specimens appearing suddenly during certain times of the year, only to die off as suddenly as they appeared. Patients may present with numerous linear red stings. Treatment of these stings is identical to treatment of other jellyfish stings: hot water, acetic acid, and sodium hydroxide paste.44,45
Figure 3. Portuguese Man O’War |
![]() |
Source: Fernando Losada Rodríguez, CC BY-SA 4.0 https://creativecommons.org/licenses/by-sa/4.0, via Wikimedia Commons |
Venomous Fish
In the waters surrounding North America, several aquatic vertebrates can cause envenomation. Stingrays and the Scorpaenidae family, including lionfish, stonefish, and catfish of the order Nematognathi, all possess poisonous barbs. Humans often are envenomated when they have provoked the fish and encounter the spines, either through fishing or accidentally stepping on the organism. Once the barb has pierced the flesh, the initial pain is from the trauma caused by the stinger. In the case of stingrays, the barb can be left as a retained foreign body, but for most other fish, the barb rarely is detached from the animal, leaving a clean puncture site. The affected area should be submerged in hot water at or near 45°C to break down the heat-labile toxin. Computed tomography (CT) imaging can identify retained foreign bodies more reliably, but ultrasound also can be used with success. As with any large, dirty wound, the patient should receive tetanus prophylaxis immediately. Antibiotic prophylaxis should be considered in any severe marine injury, with doxycycline as the antibiotic of choice to cover Vibrio species and their potential for aggressive infection. Care should be taken to continually monitor the injury to ensure healing is occurring and that infection is not occurring for the patient.46,47
The Scorpaenidae family, including the invasive lionfish (see Figure 4) and other stonefish, have spines with associated venom glands. The venom is extremely dangerous and even fatal to humans, especially in children, and given a sufficient dosage. Like crotalid venoms, this venom is a cocktail of proteases and other destructive enzymatic proteins that can cause similar pain and impressive-appearing reactions. Once stung, the victim experiences erythema, ecchymoses, and swelling accompanied by extreme pain. Should a sufficient dose be experienced, systemic toxicity will develop with the dangerous symptoms of hypotension, arrhythmogenic tachycardia, and pulmonary edema. Should a patient develop these severe symptoms, there is an equine-based stonefish antivenom available, but not in all areas.48-50
Figure 4. Lionfish |
![]() |
Source: Alexander Vasenin, CC BY-SA 3.0 https://creativecommons.org/licenses/by-sa/3.0, via Wikimedia Commons |
Catfish dwell in muddy and dark waters of American rivers and lakes. Named for the large whiskers around their mouth, these fish possess spines near their mouth and their fins, which act as a defense mechanism. As mentioned earlier, stings occur when the fish is handled, resulting in an envenomation and a mechanical injury caused by the spine’s size. Envenomation causes local skin damage and pain. As with other aquatic envenomations, immersion in 45°C water for 30-90 minutes is recommended to destroy heat-labile toxins. Ultimately, the best policy when educating individuals who encounter areas where poisonous fish are present is to focus on prevention. As a preventive measure, education should focus on footwear choice when wading in high-risk areas.51-55
Conclusion
Water covers approximately 70% of the planet’s surface, creating tremendous opportunities for divers, snorkelers, and other swimmers to explore a vast and beautiful underwater world. Despite the number of people participating in it, scuba diving and other diving activities have numerous associated risks, many of which have been described in this article. Having a better understanding of the gas laws implicated during diving allows clinicians to better identify and treat diving-related injuries.
During descent, some of the most common diving injuries include those due to barotrauma, such as inner ear and dental pain, as pockets of trapped air decrease in volume. Upon ascent, if divers are not careful to exhale, they are at risk for pulmonary barotrauma potentially causing a pneumothorax, pneumomediastinum, or air embolism as air escapes into available spaces. The ascent phase also is when decompression sickness occurs. If there is not enough time for the off-gassing of nitrogen, large nitrogen gas bubbles can travel throughout the body and cause joint pain and skin changes as seen in type 1 DCS or lead to the more emergent problems of type 2 DCS, such as respiratory distress, cardiovascular collapse, or neurologic problems.
Beyond the physiologic stressors of diving, there are other environmental considerations, such as the temperature, traumatic injuries, and encounters with various sea creatures. Whether diving recreationally or professionally, being aware of potential risks ahead of time can help with implementing mitigation strategies.
Regardless of the diving-related illness/injury, a helpful resource is the Divers Alert Network (DAN) Emergency Hotline at (919)-684-9111.
REFERENCES
- Denoble P. Participation in recreational scuba diving. Jan. 14, 2016. Divers Alert Network. https://dan.org/safety-prevention/diver-safety/divers-blog/participation-in-recreational-scuba-diving/
- Butler WP. Caisson disease during the construction of the Eads and Brooklyn Bridges: A review. Undersea Hyperb Med 2004;31:445-459.
- West JB. Robert Hooke: Early respiratory physiologist, polymath, and mechanical genius. Physiology (Bethesda) 2014;29:222–233.
- Avishay DM, Tenny KM. Henry’s Law. In: StatPearls [Internet]. StatPearls Publishing. Updated Jan. 29, 2023.
- Wilmshurst P. Diving and oxygen. BMJ 1998;317:996-999.
- The Editors of the Encyclopedia Britannica. Pascal’s principle. Encyclopedia Britannica. Updated Aug. 9, 2024. https://www.britannica.com/science/Pascals-principle
- Srivastav S, Jamil RT, Zeltser R. Valsalva Maneuver. In: StatPearls [Internet]. StatPearls Publishing. Updated Aug. 21, 2023.
- Divers Alert Network. Mask squeeze (facial barotrauma). Divers Alert Network. Feb. 1, 2021. https://dan.org/health-medicine/health-resources/diseases-conditions/mask-squeeze-aka-facial-barotrauma/
- Walker JR III, Hexdall EJ, Murphy-Lavoie HM. Diving Gas Embolism. In: StatPearls [Internet]. Updated July 31, 2023. StatPearls Publishing.
- Moon RE. Hyperbaric treatment of air or gas embolism: Current recommendations. Undersea Hyperb Med 2019;46:673-683.
- Vaezeafshar R, Psaltis AJ, Rao VK, et al. Barosinusitis: Comprehensive review and proposed new classification system. Allergy Rhinol (Providence) 2017;8:109-117.
- Moon RE. Gas toxicity during diving. Reviewed/revised April 2023. Merck Manuals Consumer Version. https://www.merckmanuals.com/home/injuries-and-poisoning/diving-and-compressed-air-injuries/gas-toxicity-during-diving#:~:text=Oxygen%20Toxicity%20During%20Diving,reaches%201.4%20atmospheres%20or%20greater
- Cooper JS, Phuyal P, Shah N. Oxygen Toxicity. In: StatPearls [Internet]. Updated Aug. 1, 2023. StatPearls Publishing.
- Shreeves K. The ultimate guide to nitrox diving. SCUBA Diving. June 11, 2024. https://www.scubadiving.com/nitrox-scuba-diving-guide-certification
- Kirkland PJ, Mathew D, Modi P, Cooper JS. Nitrogen Narcosis in Diving. In: StatPearls [Internet]. StatPearls Publishing. Updated July 31, 2023.
- Raymond KA, Cooper JS. Scuba Diving Physiology. In: StatPearls [Internet]. Updated Oct. 10, 2022. StatPearls Publishing.
- Marinovic J, Ljubkovic M, Obad A, et al. Effects of successive air and trimix dives on human cardiovascular function. Med Sci Sports Exerc 2009;41:2207–2212.
- Vann RD, Butler FK, Mitchell SJ, Moon RE. Decompression illness. Lancet 2011;377:153-164.
- Pollock NW, Buteau D. Updates in decompression illness. Emerg Med Clin North Am 2017;35:301-319.
- Mitchell SJ, Bennett MH, Bryson P, et al. Pre-hospital management of decompression illness: Expert review of key principles and controversies. Diving Hyperb Med 2018;48:45-55.
- Krause KM, Pilmanis AA. The effectiveness of ground level oxygen treatment for altitude decompression sickness in human research subjects. Aviat Space Environ Med 2000;71:115-118.
- Moon RE, Mitchell SJ. Hyperbaric oxygen for decompression sickness. Undersea Hyperb Med 2021;48:195-203.
- Kirby JP. Hyperbaric oxygen therapy emergencies. Mo Med 2019;116:180-183.
- Navy Department. U.S. Navy Diving Manual. Rev 6 Vol 5: Diving Medicine and recompression chamber operations. NAVSEA 0910-LP-106-0957. Naval Sea Systems Command; 2008.
- Denny M. Scuba diving hand signals. (Updated by Schofield D, Nov. 5, 2023.) PADI Blog. Professional Association of Diving Instructors (PADI). https://blog.padi.com/scuba-diving-hand-signals/
- Bart RM, Lau H. Shallow Water Blackout. In: StatPearls [Internet]. Updated Jan. 30, 2023. StatPearls Publishing.
- Pothiawala S, Civil I. A rare case of penetrating intra-abdominal trauma by a fishing speargun. N Z Med J 2022;135:113-119.
- Semeraro D, Passalacqua NV, Symes S, Gilson T. Patterns of trauma induced by motorboat and ferry propellers as illustrated by three known cases from Rhode Island. J Forensic Sci 2012;57:1625-1629.
- Scalia G, Silven MP, Costanzo R, et al. Motorboat propeller-related head injuries: A systematic literature review with a case illustration. Surg Neurol Int 2023;14:175.
- Walker P. Steve Irwin could not have been saved, says witness to stingray attack. The Guardian. March 10, 2014. https://www.theguardian.com/tv-and-radio/2014/mar/10/steve-irwin-could-not-be-saved-stringray-justin-lyons
- Kosequat J, Rush SC, Simonsen I, et al. Efficacy of the mnemonic device “MARCH PAWS” as a checklist for pararescuemen during tactical field care and tactical evacuation. J Spec Oper Med 2017;17:80-84.
- Dow J, Giesbrecht GG, Danzl DF, et al. Wilderness Medical Society clinical practice guidelines for the out-of-hospital evaluation and treatment of accidental hypothermia: 2019 update. Wilderness Environ Med 2019;30:S47-S69.
- Paal P, Pasquier M, Darocha T, et al. Accidental hypothermia: 2021 Update. Int J Environ Res Public Health 2022;19:501.
- Durrer B, Brugger H, Syme D; International Commission for Mountain Emergency Medicine. The medical on-site treatment of hypothermia: ICAR-MEDCOM recommendation. High Alt Med Biol 2003;4:99-103.
- Lafère P, Guerrero F, Germonpré P, Balestra C. Comparison of insulation provided by dry or wetsuits among recreational divers during cold water immersion (< 5°C). Int Marit Health 2021;72:217-222.
- Romet TT. Mechanism of afterdrop after cold water immersion. J Appl Physiol (1985) 1988;65:1535-1538.
- Giesbrecht GG. Cold stress, near drowning and accidental hypothermia: A review. Aviat Space Environ Med 2000;71:733-752.
- Hultzer MV, Xu X, Marrao C, et al. Pre-hospital torso-warming modalities for severe hypothermia: A comparative study using a human model. CJEM 2005;7:378-386.
- Avellanas Chavala ML, Ayala Gallardo M, Soteras Martínez Í, Subirats Bayego E. Management of accidental hypothermia: A narrative review. Med Intensiva (Engl Ed) 2019;43:556-568.
- Gilardi E, Petrucci M, Sabia L, et al. High-flow nasal cannula for body rewarming in hypothermia. Crit Care 2020;24:122.
- Lakkis NA, Maalouf GJ, Mahmassani DM. Jellyfish stings: A practical approach. Wilderness Environ Med 2015;26:422-429.
- Kong EL, Nappe TM. Irukandji syndrome. In: StatPearls [Internet]. Updated Aug. 14, 2023. StatPearls Publishing.
- Cegolon L, Heymann WC, Lange JH, Mastrangelo G. Jellyfish stings and their management: A review. Mar Drugs 2013;11:523-550.
- Currie BJ. Marine antivenoms. J Toxicol Clin Toxicol 2003;41:301-308.
- Kaufman MB. Portuguese man-of-war envenomation. Pediatr Emerg Care 1992;8:27-28.
- Charnigo A, Thiele G, Kong EL, Ondrus RJ. Stingray Sting. In: StatPearls [Internet]. Updated July 17, 2023. StatPearls Publishing.
- Clark RF, Girard RH, Rao D, et al. Stingray envenomation: A retrospective review of clinical presentation and treatment in 119 cases. J Emerg Med 2007;33:33-37.
- Meyer PK. Stingray injuries. Wilderness Environ Med 1997;8:24-28.
- Rensch G, Murphy-Lavoie HM. Lionfish, Scorpionfish, and Stonefish Toxicity. In StatPearls. Updated July 31, 2023. StatPearls Publishing.
- Hornbeak KB, Auerbach PS. Marine envenomation. Emerg Med Clin North Am 2017;35:321-337.
- Haddad V Jr, Lupi O, Lonza JP, Tyring SK. Tropical dermatology: Marine and aquatic dermatology. J Am Acad Dermatol 2009;61:733-750; quiz 751-752.
- Blomkalns AL, Otten EJ. Catfish spine envenomation: A case report and literature review. Wilderness Environ Med 1999;10:242-246.
- Satora L, Pach J, Targosz D, Szkolnicka B. Stinging catfish poisoning. Clin Toxicol (Phila) 2005;43:893-894.
- Scoggin CH. Catfish stings. JAMA 1975;231:176-177.
- Junqueira MEP, Grund LZ, Orii NM, et al. Analysis of the inflammatory reaction induced by the catfish (Cathorops spixii) venoms. Toxicon 2007;49:909-919.
This article describes the various gas laws that govern underwater physiology and common underwater trauma and mishaps.
Subscribe Now for Access
You have reached your article limit for the month. We hope you found our articles both enjoyable and insightful. For information on new subscriptions, product trials, alternative billing arrangements or group and site discounts please call 800-688-2421. We look forward to having you as a long-term member of the Relias Media community.